Muscle tissue has a unique histological appearance which enables it to carry out its function. There are three main types of muscle:
- Skeletal – striated muscle that is under voluntary control from the somatic nervous system. Identifying features are cylindrical cells and multiple peripheral nuclei.
- Cardiac – striated muscle that is found only in the heart. Identifying features are single nuclei and the presence of intercalated discs between the cells.
- Smooth – non-striated muscle that is controlled involuntarily by the autonomic nervous system. The identifying feature is the presence of one spindle-shaped central nucleus per cell.
In this article, we will look at the histology of skeletal muscle – its composition, histological appearance and clinical correlations.
Composition of Skeletal Muscle
A muscle cell is very specialised for its purpose. A single cell forms one muscle fibre, and its cell surface membrane is known as the sarcolemma.
T tubules are unique to muscle cells. These are invaginations of the sarcolemma that conduct charge when the cell is depolarised.
Muscle cells also have a specialised endoplasmic reticulum – this is known as the sarcoplasmic reticulum and contains a large store of calcium ions.
Muscles also have an intricate support structure of connective tissue. Each muscle fibre is surrounded by a thin layer of connective tissue known as endomysium. These fibres are then grouped into bundles known as fascicles, which are surrounded by a layer of connective tissue known as perimysium. Many fascicles make up a muscle, which in turn is surrounded by a thick layer of connective tissue known as the epimysium.
Ultrastructural Appearance of Skeletal Muscle
The striated appearance of skeletal muscle fibres is due to the organisation of two contractile proteins: actin (thin filament) and myosin (thick filament).
The functional unit of contraction in a skeletal muscle fibre is the sarcomere, which runs from Z line to Z line. A sarcomere is broken down into a number of sections:
- Z line – where the actin filaments are anchored.
- M line – where the myosin filaments are anchored.
- I band – contains only actin filaments.
- A band – the length of a myosin filament, may contain overlapping actin filaments.
- H zone – contains only myosin filaments.
A useful acronym is MHAZI – the M line is inside the H zone which is inside the A band, whilst the Z line is inside the I band.
Sliding Filament Model
The sliding filament model describes the mechanism of skeletal muscle contraction
Actin and Myosin
Muscle fibres are formed from two contractile proteins – actin and myosin.
Myosin filaments have many heads, which can bind to sites on the actin filament. Actin filaments are associated with two other regulatory proteins, troponin and tropomyosin. Tropomyosin is a long protein that runs along the actin filament and blocks the myosin head binding sites.
Troponin is a small protein that binds the tropomyosin to the actin. It is made up of three parts:
- Troponin I – binds to the actin filament.
- Troponin T – binds to tropomyosin.
- Troponin C – can bind calcium ions.
Excitation-Contraction Coupling
The unique structure of troponin is the basis of excitation-contraction coupling:
- When depolarisation occurs at a neuromuscular junction, this is conducted down the t-tubules, causing a huge influx of calcium ions into the sarcoplasm from the sarcoplasmic reticulum.
- This calcium binds to troponin C, causing a change in conformation that moves tropomyosin away from the myosin head binding sites of the actin filaments.
- This allows the myosin head to bind to the actin, forming a cross-link. The power stroke then occurs as the myosin heads pivots in a ‘rowing motion’, moving the actin past the myosin towards the M line.
- ATP then binds to the myosin head, causing it to uncouple from the actin and allowing the process to repeat.
Hence in contraction, the length of the filaments does not change. However, the length of the sarcomere decreases due to the actin filaments sliding over the myosin. The H zone and I band shorten, whilst the A band stays the same length. This brings the Z lines closer together and causes overall length of the sarcomere to decrease.
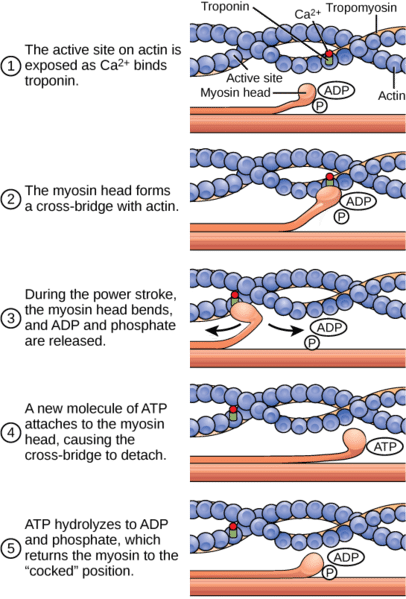
Fig 2 – The sliding filament model of muscle contraction.
Clinical Relevance – Duchenne Muscular Dystrophy
Duchenne muscular dystrophy is a a recessive X-linked genetic disorder in which dystrophin, a protein which anchors the sarcolemma to the myofilaments, is not produced.
This leads to the muscle fibres tearing themselves apart on contraction, causing progressive muscle weakness and wasting.
It has an early onset, with patients often being wheelchair-dependent by the age of 12.